In the first paper, Thiamine Deficiency in Modern Medical Practice , I provided an overview of why health practitioners should consider thiamine in general practice. In this paper, I would like to delve more deeply into how one becomes deficient in the 21st century.
Thiamine and Its RDA
Thiamine, or vitamin B1, is an essential and rate limiting nutrient required for metabolic health. Like the other B vitamins, it is water-soluble. Unlike some other B vitamins, it has a very short half-life (1-12 hours), and a limited reserve of about 30 milligrams. Absent regular consumption, deficiency arises quickly, manifesting symptoms that range from general fatigue, mood lability, anorexia, and nausea to cardiac irregularities, neuromuscular and neurocognitive deficits. In developed countries, where food enrichment and fortification programs have added thiamine to grain and other products, thiamine deficiency syndromes are considered to be rare and largely confined to specific populations and circumstances where thiamine ingestion, absorption, metabolism, or excretion are impaired such as poverty-based malnutrition, alcoholism, severe gut dysbiosis and/or hyperemesis.
The recommended daily allowance (RDA) put forth by health institutions considers 1.1-1.2mg of thiamine sufficient for most adults to stave off deficiency. This requirement is met easily with any modern diet, even a poor one, suggesting that the suspected low incidence of deficiency is accurate. And yet, across multiple studies that have measured thiamine status in different patient populations, none of whom can be considered malnourished by RDA standards, or alcoholic, the rate of deficiency is found to be between 20-98%; a discordance that suggests both institutional designations of thiamine sufficiency and deficiency are underestimated.
Insofar as thiamine is absolutely requisite for the conversion of food into cellular energy, e.g. ATP, and sufficient ATP is fundamental to metabolic health, something that has become an increasingly rare phenomenon in the Western world, it is possible that our understanding of thiamine sufficiency and deficiency is mismatched to the demands of modern living. If this is the case, then insufficient thiamine may be a key factor in many of the disease processes that plague modern medicine. Indeed, thiamine insufficiency and frank deficiency has been observed with obesity, diabetes, heart disease, gastrointestinal dysbiosis and dysmotility syndromes, post gastric bypass surgery, in cancer, Alzheimer’s, Parkinson’s, and psychiatric patients. Combined, these patient populations represent a far larger percentage of the population than recognized within the current paradigm. From this perspective, it is conceivable that the older designations of sufficiency and deficiency no longer apply and that for the 21st century patient, thiamine stability is a much more fragile endeavor than recognized.
Micronutrients and Cellular Energy
The most fundamental process to health and survival involves the conversion of consumed nutrients into ATP. Absent adequate ATP, health is impossible. Energy metabolism requires a ready supply of macronutrients (carbohydrate, protein, and fats) and at least 22 micronutrients or vitamins and minerals (see Figure 1.).
In developed countries, macronutrients are readily available, often in excess. Micronutrient intake, however, is inconsistent. A review article from the University of Oregon report found that a large percentage of the population had inadequate micronutrient status (4-65% depending upon the nutrient) despite excessive caloric intake. Moreover, much of the supposed nutrient sufficiency came from enriched or fortified foods. In other words, absent food enrichment or fortification, most children, adolescents, and adults had insufficient micronutrient intake. Inasmuch as most fortified foods come with a high caloric content, which effectively demands a higher micronutrient content to metabolize it; this presents a problem.
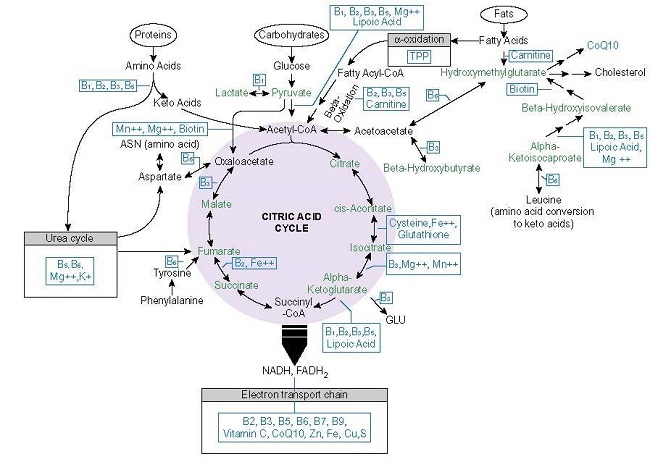
Thiamine Dependent Enzymes
From the graphic above, note how many times thiamine (vitamin B1 or TPP) appears. Thiamine is required for the transketolase (TKT), pyruvate dehydrogenase complex of enzymes (PDC), branched chain keto acid dehydrogenase (BCKAD), 2-Hydroxyacyl-CoA lyase (HACL), alpha-ketoglutarate dehydrogenase ([a-KDGH] – also called 2-oxoglutarate dehydrogenase complex [OGDC]) and for lactate recycling as a cofactor for the lactate dehydrogenase complex (LDH). Beyond its coenzyme role, thiamine allosterically regulates the expression and activity other mitochondrial proteins including:
- Succinate thiokinase/succinyl-CoA synthetase: together with a-KDGH catalyzes succinyl-CoA to succinate.
- Succinate dehydrogenase: oxidizes succinate to fumarate, uses the electrons generated to catalyze reduction of ubiquinone to ubiquinol for complex II (TCA>ETC linkage)
- Malate dehydrogenase (MDH): interconversion of malate and oxaloacetate with cofactor NAD+ or NADP+.
- Pyridoxal kinase: converts dietary vitamin B6 into the active cofactor form pyridoxal 5′-phosphate (PLP) creating a functional deficiency.
With low or absent thiamine, each of these enzymes downregulates from 10% to almost 30% resulting in a reduction of ATP from 38 to ~13 units (in culture).
Thiamine Is Fundamental
Among the 22 micronutrients needed to convert macronutrient ATP, thiamine, along with its cofactor, magnesium, sit at the entry points to this process. That means that thiamine availability controls the rates of carbohydrate, protein, and fat metabolism and their subsequent conversion into ATP. Insufficient thiamine, even marginally so, impedes this process resulting in not only reduced ATP, but also, impaired cellular respiration, and increased oxidative stress and advanced glycation end products (AGEs); the very cascades linked to the preponderance of modern diseases dominating the healthcare landscape.
- Cellular respiration, the ability to use molecular oxygen, requires ATP, which requires thiamine. Insufficient thiamine causes cell level hypoxia and upregulates the expression of hypoxia inducible factors (HIFs). HIFs are responsible for oxygen homeostasis, regulating at least 100 other proteins including those involved in angiogenesis, erythropoiesis and iron metabolism, glucose metabolism, growth factors, and apoptosis. HIF stabilization is implicated in a range of illnesses from autoimmune disease, to heart disease and cancer.
- Reactive oxygen species (ROS) are a natural byproduct of ATP production and serve as useful mitochondrial signaling agents. Elevated ROS, relative to antioxidant capacity, however, creates oxidative stress, damaging cellular lipids, proteins and DNA. Antioxidant capacity is reduced with thiamine deficiency while ROS are increased.
- AGEs, the toxic byproducts of hyperglycemia and oxidative stress, are modulated by thiamine. With sufficient thiamine, AGE precursors are shunted towards energy metabolism via the transketolase and the pentose phosphate pathway rather than accumulating in tissue as reactive carbonyl intermediates common with metabolic disease.
Each of these play a role in the pathophysiology of diabetes, cardiovascular and neurodegenerative diseases. This makes thiamine status, by way of its role in ATP production, cell respiration, ROS management, and AGE metabolism, a critical variable determining health or disease.
Given its position and role in these processes, it is not difficult to imagine how insufficient thiamine intake might derange and diminish energy metabolism and how that, in turn, might impact metabolic health both locally at the cell, tissue and organ level, and systemically. What is difficult to imagine, however, given the miniscule RDA requirement for a little over a single milligram of thiamine, is how anyone in the developed world where food scarcity is rare, where thiamine is readily available in both whole foods and in fortified foods, becomes thiamine deficient. And yet, a growing body of research suggests that is exactly what is happening. Recall from above, that depending upon the population studied, insufficient thiamine to frank deficiency has been found in 20-98% of the patients tested.
Modern Challenges to Thiamine Sufficiency From Consumption to Utilization
As an essential nutrient, thiamine must be consumed from foods, absorbed, activated and transported to where it is needed, and then utilized by its cognate enzymes. At each of these steps there are challenges that diminish thiamine availability, effectively increasing thiamine need well beyond the current RDA values. In fact, many of the products and amenities that make modern living what it is, imperil thiamine status and do so at multiple junctions. The additive effects of these challenges leaves many vulnerable to deficiency.
Dietary Sources of Thiamine
The highest concentrations of thiamine in natural and non-manufactured foods come from pork, fish (salmon, trout, tuna, catfish), many nuts and seeds (macadamia, pistachios, sunflower seeds, flax seed), beans (navy, black, black-eyed peas, lentils), peas, tofu, brown rice, whole wheat, acorn squash, asparagus, and many other foods. A diet rich in organic, whole foods is generally sufficient to meet the daily requirements for the thiamine and other vitamins and minerals. Likewise, though less ideal, a diet of processed foods that has been enriched or fortified with thiamine, will meet the RDA for thiamine quite easily, perhaps even exceed it. Indeed, one serving of breakfast cereal is sufficient to reach the RDA for thiamine.
Despite the ready availability of thiamine in both whole and processed foods, the data suggest that many people find it difficult to maintain thiamine status. This is due to the interactions between the endogenous chemistry of thiamine metabolism and the chemistry of exogenous variables affecting thiamine stability. The most common factors affecting thiamine status, include high calorie, high toxicant load diets, alcohol and/or tobacco use, caffeine products, and pharmaceutical and chemical exposures.
Dietary Impediments to Thiamine Sufficiency
While fortification provides access to thiamine, highly processed foods carry a high calorie and toxicant count making them metabolically deleterious despite any potential gains from vitamin enrichment or fortification. High carbohydrate, highly processed foods diminish thiamine status by multiple mechanisms.
- Carbohydrate ingestion requires thiamine for oxidation. When more than 55% of total caloric intake comes from carbohydrates, no matter their source, thiamine status in otherwise healthy and thiamine sufficient individuals declines. In contrast, a lower carbohydrate higher fat diet slows thiamine loss in thiamine-restricted experimental conditions, while protein seems to preserve thiamine degradation in foods.
- Diets high in fructose are linked to the endogenous production of oxythiamine, an anti-thiamine molecule.
- From farm to plate, every aspect of commercial food production involves the usage of chemical products that are toxic to the mitochondria and to thiamine status, making consumption of these types of foods a net loss metabolically.
Other common dietary contributors to insufficient thiamine.
- Alcohol, when ingested with thiamine rich foods, blocks conversion of dietary thiamine into active thiamine, reducing thiamine availability by as much as 54%. If consumed in sufficient quantities with regularity, it damages the intestinal mucosa leading to impaired absorption and dysbiosis.
- The nicotine in tobacco products inhibits thiamine transporter mediated uptake in the pancreatic acinar cells by >40%. Deficiency reduces insulin secretion. In combination with alcohol ingestion, smoking is implicated in the development of pancreatitis.
- The caffeic acid, chlorogenic acid, and tannic acid in coffee, tea, and energy drinks, oxidize the thiazole ring of the thiamine molecule, impairing its absorption, while the added sugars, flavors and other substances to enhance taste, increase thiamine demand.
Although food scarcity is not as prevalent in developed countries compared to undeveloped regions, poverty still impacts nutrient status. This owes largely to the fact that highly processed foods, high calorie foods are less expensive than whole foods and thus, there is an over-reliance on carbohydrate consumption to meet caloric requirements. Here, obesity and metabolic dysfunction co-occur with micro-nutrient and sometimes macronutrient, e.g. protein, deficiency.
Pharmaceutical and Environmental Threats to Thiamine Status
After high calorie malnutrition and other dietary habits that limit thiamine availability, the next most common threat to thiamine sufficiency is the use of pharmaceuticals. This variable cannot be stressed enough. Pharmaceutical chemicals deplete thiamine and other nutrients, directly or indirectly by a number of mechanisms. Some of this is by design, such as with antibiotics that target folate and thiamine, some of it represents off-target effects, such as the blockade of thiamine transporters by metformin and the other 146 drugs tested for this action, an increase in demand in order to withstand other mitochondrial damage. Regardless of the intended purpose, however, pharmaceuticals represent chemical stressors to thiamine and nutrient stability. As such, their regular use necessitates a concerted approach to maintain nutrient status. Some of the most commonly used medications are the biggest offenders:
- Metformin
- Antibiotics
- NSAIDs, acetaminophen, and aspirin
- Proton pump inhibitors
- Psychiatric medications
- Diuretics
- Chemotherapy
In addition to the ingestion of pharmaceutical chemicals, environmental chemical exposures damage mitochondrial functioning, even at low, and what are considered, non-toxic exposures. These exposures are pervasive, often unavoidable, and tend to accrue over time, with additive and synergistic effects to other stressors. Consider the totality of a patient’s toxic load when addressing the risk of nutrient insufficiency.
Absorption and Metabolism
Assuming sufficient thiamine is ingested from diet and is not blocked or otherwise degraded by food, pharmaceutical or environmental chemicals, it then has to be absorbed in the intestines before it can be activated and transported to organs and tissues for use. Epithelial injury, microbial dysbiosis, and genetic variation, all of which are common, limit the effectiveness of this phase. Epithelial injury and microbial dysbiosis slow passive absorption, while genetic, epigenetic, and environmental variables, slow or block active transport.
At low concentrations, thiamine is absorbed in the small intestine by active transport, while higher concentrations are absorbed by passive diffusion. Active transport is mediated by two primary thiamine transporters, ThTR1 and ThTR2, and a number of additional transporters that fall under the solute carrier family of genes:
- SLC19A1: folate transporter, but also, transports thiamine mono- and di- phospho derivatives.
- SLC19A2 (ThTr1): systemic thiamine transport, main transporter in pancreatic islet tissue and hematopoietic cells; most abundant, from highest to lowest in the intestine, skeletal muscle, nervous system, eye, placenta, liver, and kidney.
- SLC19A3 (ThTr2): primary intestinal thiamine transporter, also located in adipose tissue, breast tissue, liver, lymphocytes, spleen, gallbladder, placenta, pancreas, and brain.
- SLC22A1 (OCT1): organic cation transporter 1, primary hepatic thiamine transporter; competitively inhibited with transport of metformin, xenobiotics, and other drugs.
- SLC25A19 (MTPP-1): mitochondrial thiamine pyrophosphate carrier.
- SLC35F3: endoplasmic reticulum and Golgi thiamine transporter, implicated in hypertension.
- SLC44A4 (hTPPT/TPPT-1): absorption of microbiota-generated thiamine pyrophosphate in the large intestine.
Although conventional wisdom suggests that only homozygous mutations affect the performance of these proteins, in reality, there is a gradation of abnormalities that challenge thiamine uptake, particularly when environmental or pharmaceutical variables block or otherwise limit the functioning of the same protein. In some cases, genetic difficulties can be compensated for providing nutrient support at supraphyisiological doses, among the better known examples:
- Thiamine responsive megaloblastic anemia (mutations in SLC19A2/ThTr1); megaloblastic anemia, progressive sensorineural hearing loss, and diabetes mellitus.
- Biotin-thiamine responsive basal ganglia disease (mutations in SLC19A3/ThTr 2) presents in infancy or childhood with recurrent subacute encephalopathy, confusion, seizures, ataxia, dystonia, supranuclear facial palsy, external ophthalmoplegia, and/or dysphagia or Leigh-like syndrome with infantile spasms. When presenting in adulthood, acute onset seizures, ataxia, nystagmus, diplopia, and ophthalmoplegia.
- Thiamine responsive Leigh Syndrome (mutations in in the SLC19A3/ThTr2).
- Thiamine metabolism dysfunction syndrome-4 (mutations SLC25A19/MTPP-1); episodic encephalopathy and febrile illness, transient neurologic dysfunction, and a slowly progressive axonal polyneuropathy.
- Thiamine Pyrophosphokinase 1 (TPL1) defects cause problems in the activation of free thiamine to thiamine pyrophosphate, rendering much of the thiamine consumed unusable. TPK1 defects have been identified as condition called thiamine metabolism dysfunction syndrome 5 or Leigh-like syndrome because of the similarity in symptoms. More recently, TPK1 defects have been found associated with Huntington’s disease. High dose thiamine appears to overcome the defect in some cases.
Thiamine Activation/Deactivation
Before it can be used, free thiamine has to be phosphorylated into its active form thiamine pyrophosphate (TPP), also called thiamine diphosphate (ThDP/TDP). This is done by the enzyme thiamine pyrophosphokinase (thiamine diphosphokinase), which is magnesium dependent and requires ATP. Magnesium deficiency is common in developed countries. TPP accounts for almost 90% of circulating thiamine.
Additional thiamine metabolites include thiamine monophosphate (TMP) and thiamine triphosphate (TTP) along with the recently discovered adenosine thiamine triphosphate (AThTP) and adenosine thiamine diphosphate (AThDP). AThTP and AThDP are produced by E.coli during periods of nutrient starvation and have been found in most mammalian tissue. This likely represents a salvage pathway common in many pathogenic microbes.
Microbial Thiamine Synthesis
It is important to note, that although the consumption of dietary thiamine provides the main sources of this nutrient systemically, a smaller, but notable (2.3%), percentage of thiamine and other B vitamins is produced endogenously by various commensal bacterial populations in both the small and large intestines. At least 10 species of bacteria synthesize thiamine that is absorbed and utilized by the colonocytes. Endogenous thiamine synthesis is reduced by diets high in simple carbohydrates but increased with complex carbohydrates. Antibiotics and other medications inhibit endogenous synthesis of B vitamins directly by design as in the case trimethoprim and sulfamethoxazole and indirectly via additional that disrupt thiamine availability. Additionally, a number of pathogenic microbes produce enzymes that degrade bacterially produced thiamine suggesting the balance of gut biota is influenced by and influences nutrient availability.
In the large intestine, bacterially synthesized TPP is absorbed directly into the colon via a population of TTP transporters (TPPT-1) in the apical membrane and then transported directly into the mitochondria via the MTPP-1 for ATP production. The reduction of colonocyte thiamine and thus ATP, would force a shift towards the more pathogenic microbial populations that thrive in nutrient deficient environments and dysregulate bowel motility. This local thiamine deficiency may be a contributing factor in large bowel microbial virulence and the dysmotility syndromes so common in modern medical practice.
Enzyme Activation
The final step in attaining thiamine sufficiency is utilization. Returning to Figure 1., the key enzymes involved in this process include: TKT, PDC, HACL, BCKAD, a-KGDH and LDH. This is an addition to the enzymes involved in the phosphorylation of free thiamine and the remaining enzymes in the Krebs cycle whose gene expression depends upon thiamine status. As with the variances and mutations in the transporters, supraphyisiological doses of thiamine may compensate for decrements in enzyme function. This has been observed in thiamine responsive PDC deficiency, characterized by excessive lactic acid; and in maple syrup urine disease, where mutations in the thiamine dependent BCKAD enzyme responsible for amino acid metabolism is impaired; also in Leigh-like syndrome, where mutations in TPK1 enzyme, which converts free thiamine to active TPP, is affected.
Is the Thiamine RDA Sufficient?
Both the chemistry and the data suggest that the current RDA of just a single milligram of thiamine is insufficient to meet the challenges presented by modern diets and chemical exposures. Owing to its role in energy metabolism, thiamine insufficiency may underlie many of the disease processes associated with metabolic dysfunction, where cellular hypoxia, increased ROS and AGEs are present. These disease processes develop long before, and sometimes absent, frank deficiency suggesting there may be gradations of insufficiency relative to the individual’s metabolic needs. Whether thiamine is a causative variable in these disease processes or simply a consequence of a complicated history of negative interactions between genetics, diet, and exposures is unclear. What is clear, however, is that thiamine insufficiency is likely far more prevalent than recognized and given its role in energy metabolism, ought to be addressed more consistently in clinical care.
We Need Your Help
More people than ever are reading Hormones Matter, a testament to the need for independent voices in health and medicine. We are not funded and accept limited advertising. Unlike many health sites, we don’t force you to purchase a subscription. We believe health information should be open to all. If you read Hormones Matter, like it, please help support it. Contribute now.
Yes, I would like to support Hormones Matter.
What are the specific 22 nutrients of metabolism?
Dear Mrs. Marrs,
First of all thank you for your work and the work of Mr. Lonsdale. Since March I have gone through a horror
Story it seems I have had a serve thiamine deficiency. I had several strange symptoms including almost completely energy lost in body. My practioner gave me the advice to take benfothiamine. This helped me a lot. At the beginning I had to take every 5-8 h one 150mg benfo otherwise I had the feeling I will collapse and the foot isn’t getting energy in my body. There was no fuel anymore in my brain or cns. I have had also neurological issues. Walking normal distances was not possible my cns felt out of fuel. I have read a lot of stuff about thiamine deficiency. Currently I am still taking b complex, magnesium 250mg and 150-300mg benfothiaine per day. What is really strange is that I have a metallic taste in my mouth this is coming from my upper jaw. Sometimes better sometimes more worse. No doctor here could help me really. I have done some blood tests about toxication and minerals, vitamins. I am awaiting the results. Long story short: do you have any ideas regarding the metallic taste? If this is happening more heavy I am also feeling sick and strange. Thank you in advance. Ben